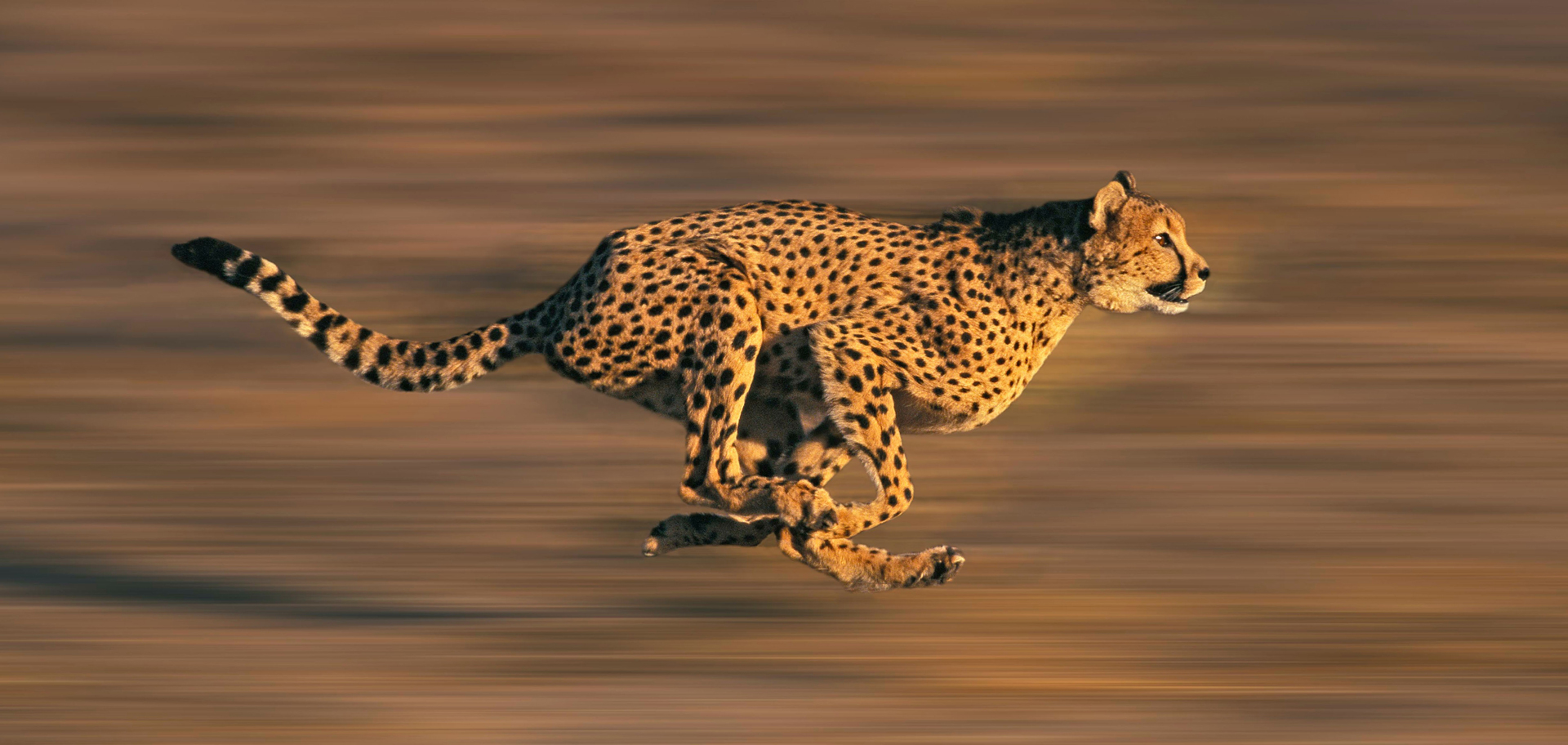
Medium-sized animals—around 110 pounds—exist in a physical sweet spot. They are the fastest. Cheetahs, for example, can reach speeds of up to 65 miles per hour.
You’ve no doubt heard the phrase “size matters,” used today quasi-humorously in almost every imaginable context in which the size of a particular object—animate or inanimate—is discussed.
For example, one ESPN Classic article by writer Mike Puma on the racehorse Seabiscuit was titled Size Doesn’t Matter. The famous horse was once considered an undersized runt with little chance of winning against champions, despite his ancestry as the grandson of Man o’ War, widely regarded as one of the greatest racehorses of all time. When Seabiscuit beat the reigning champion, War Admiral, in 1938, his place in history and legend was secured.
So, sometimes size matters, but sometimes it doesn’t.
How about in nature, does size truly matter?
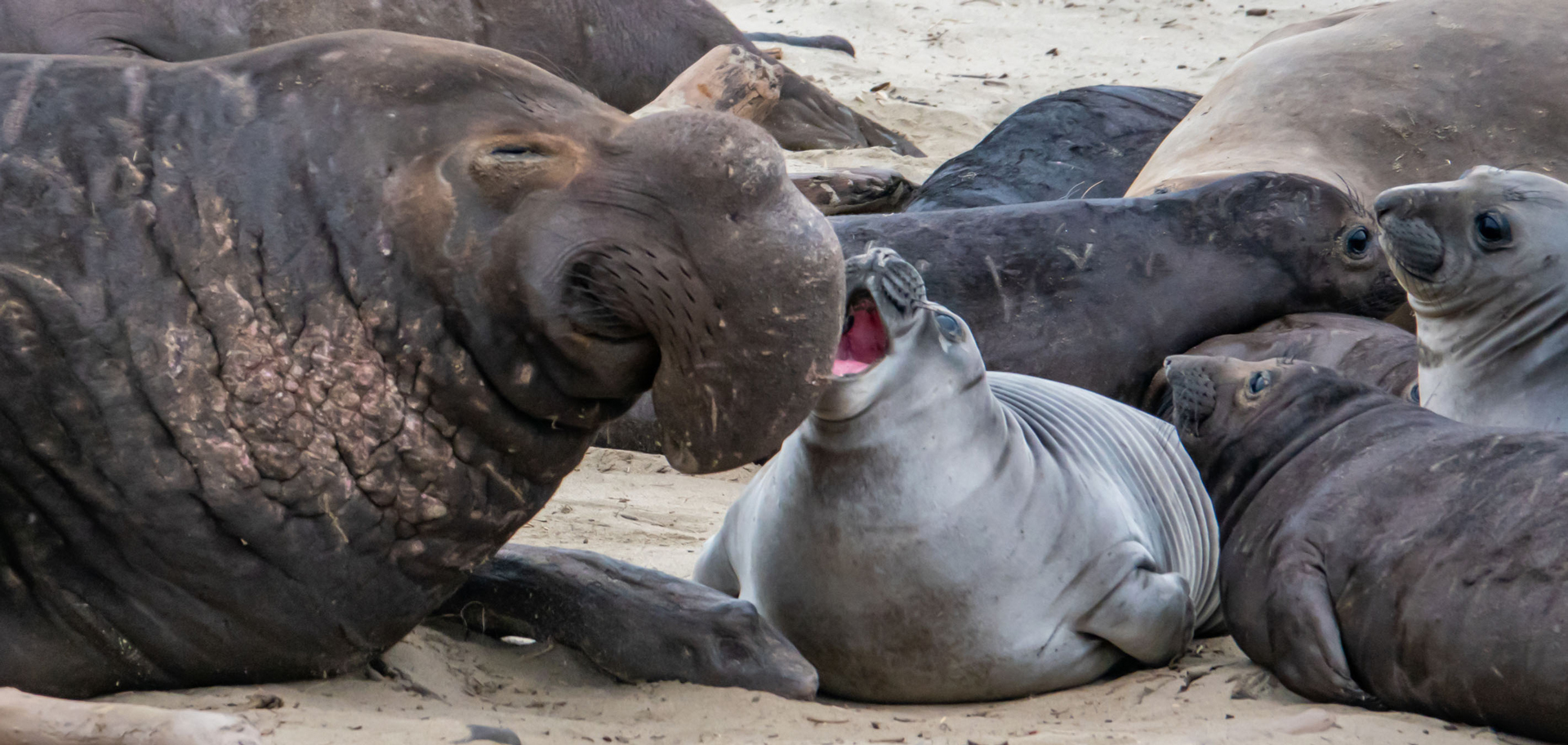
Elephant seals are characterized by a trait known as “sexual size dimorphism.” The males are around three times bigger than the females.
For mammals with more complex brains, size doesn’t matter
In many mammal species, the males are typically bigger than the females (or vice versa), a trait called sexual size dimorphism (SSD). For example, male elephant seals are about three times bigger than female elephant seals. In contrast, dolphins show no difference in size between the sexes. Humans fall somewhere in between, with the average male being larger than the average female; but across the population, there is an overlap.
Defined simply, a genome is the complete set of DNA (genetic material) in an organism. To understand how SSD is associated with genome evolution (the process by which a genome changes in structure or size over time), scientists from England’s Milner Center for Evolution at the University of Bath looked at similarities between the genomes of 124 species of mammals, grouping the genes into families of similar functions and measuring the size of these gene families.
They found that those species with a big difference in size between the sexes had bigger gene families linked to olfactory (sense of smell) function and smaller gene families associated with brain development. Therefore, this could also mean that those species with very little difference in sizes between females and males (termed monomorphic) had bigger gene families associated with brain development.
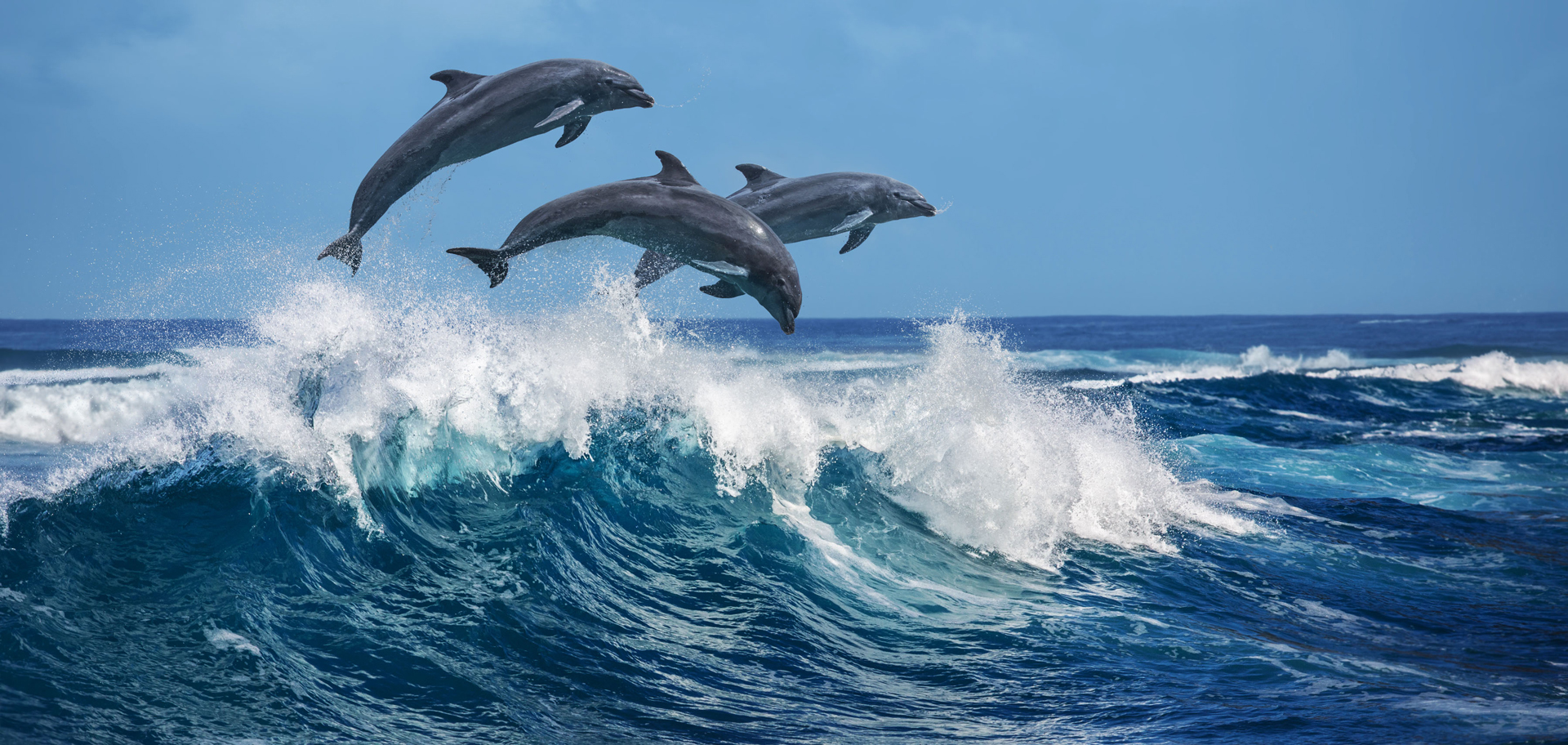
In contrast, dolphins have no difference in size between the sexes.
Publishing their results in the science journal Nature Communications in July 2024, the University of Bath scientists state that they were surprised to see such a strong statistical link between a large SSD and expanded gene families for olfactory function, which could be important for identifying mates and territories. Even more interesting, they say, is that mammals with a smaller SSD are potentially investing in their brain development and tend to have more complex social structures, such as biparental care and monogamous breeding systems. This means that they compete for mates using methods other than simply size.
For cheetahs, a sweet-spot size explains their unrivaled speed
Here’s an odd fact: while many key traits in the animal kingdom—such as brain size, lifespan, limb length and strength—tend to increase with size, maximum running speeds tend to be greatest in medium-sized animals. For instance, the fastest animals are neither large elephants nor tiny ants, but those intermediately sized, such as cheetahs. Why does running speed break with the regular patterns that govern most other aspects of animal anatomy and performance?
To explore that question, an international team of researchers, including some from Harvard University and the University of Queensland in Australia, developed a physical model of how muscles—the universal animal motor—set limits on land animals’ top running speeds. Their findings, published in Nature Communications in March 2024, suggest that there is not one limit to maximum running speed as previously thought, but two: how fast versus by how far muscles contract. The maximum speed an animal can reach is determined by whichever limit is reached first, and that limit is dictated by an animal’s size. The key to their model, say the scientists, is understanding that maximum running speed is constrained both by how fast muscles contract, as well as by how much they can shorten during a contraction.
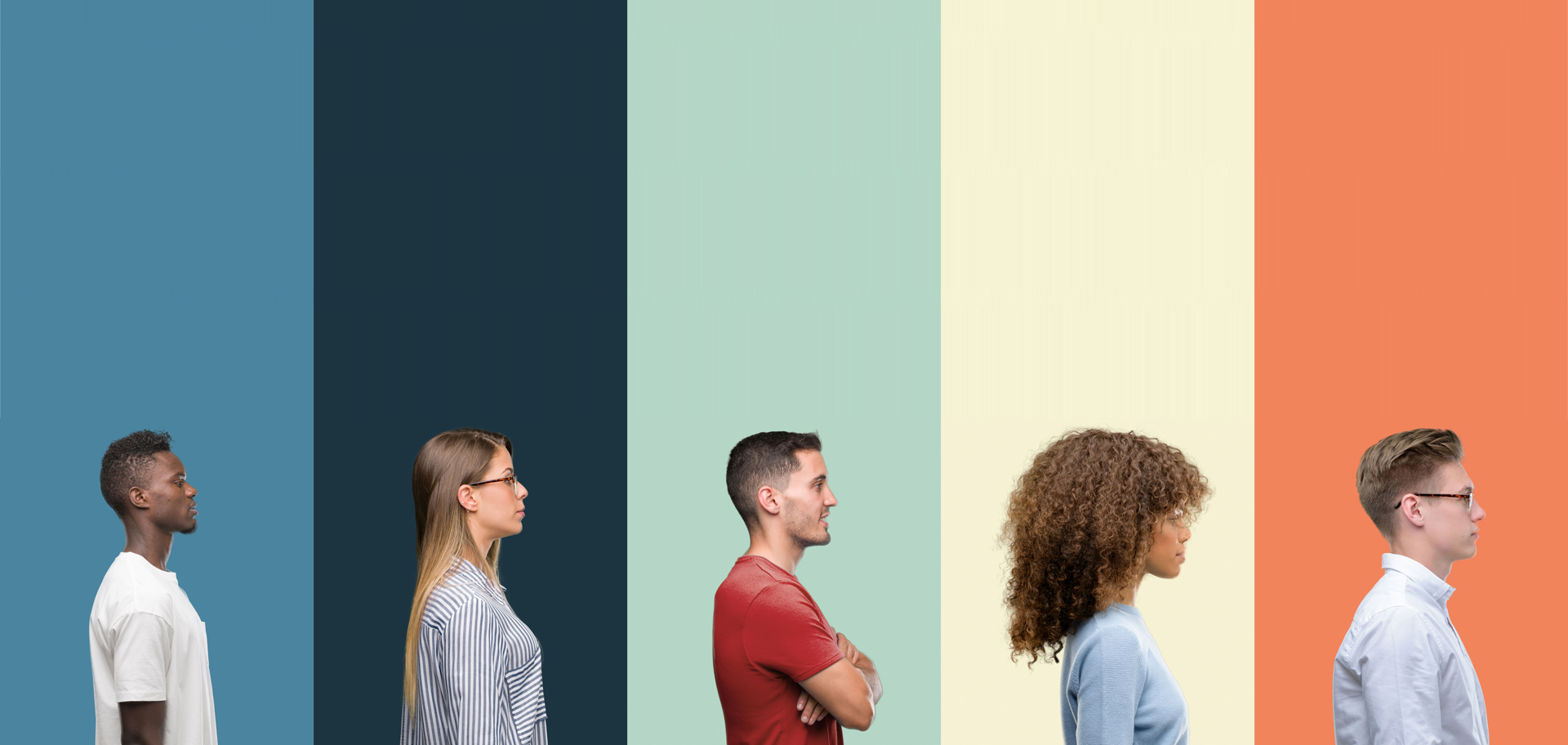
Humans, however, fall somewhere in between, with the average male being larger than the average female. There is overlap, however, across the population.
The first limit (how fast muscles contract), termed the kinetic energy capacity limit, suggests that the muscles of smaller animals are restrained by how quickly they can contract. Because small animals generate large forces relative to their weight, running for a small animal is a bit like trying to accelerate in a low gear when cycling downhill.
The second limit (how far muscles contract), called the work capacity limit, suggests that the muscles of larger animals are restrained by how far their muscles can contract. Because large animals are heavier, their muscles produce less force in relation to their weight, and running is more akin to trying to accelerate when cycling up a hill in a high gear. For instance, for large animals like elephants or rhinos, running might feel like lifting an enormous weight because their muscles are relatively weaker, and gravity demands a larger cost. As a result, animals eventually must slow down as they get bigger.
To test the accuracy of their model, the team of researchers compared its predictions to data on land-animal speed and size collected from more than 400 species, from large birds, lizards and mammals to tiny insects and spiders. The model accurately predicted how maximum running speeds vary with body size for animals that differ by more than 10 orders of magnitude in body mass, from tiny, 0.1-milligram mites to six-ton elephants.
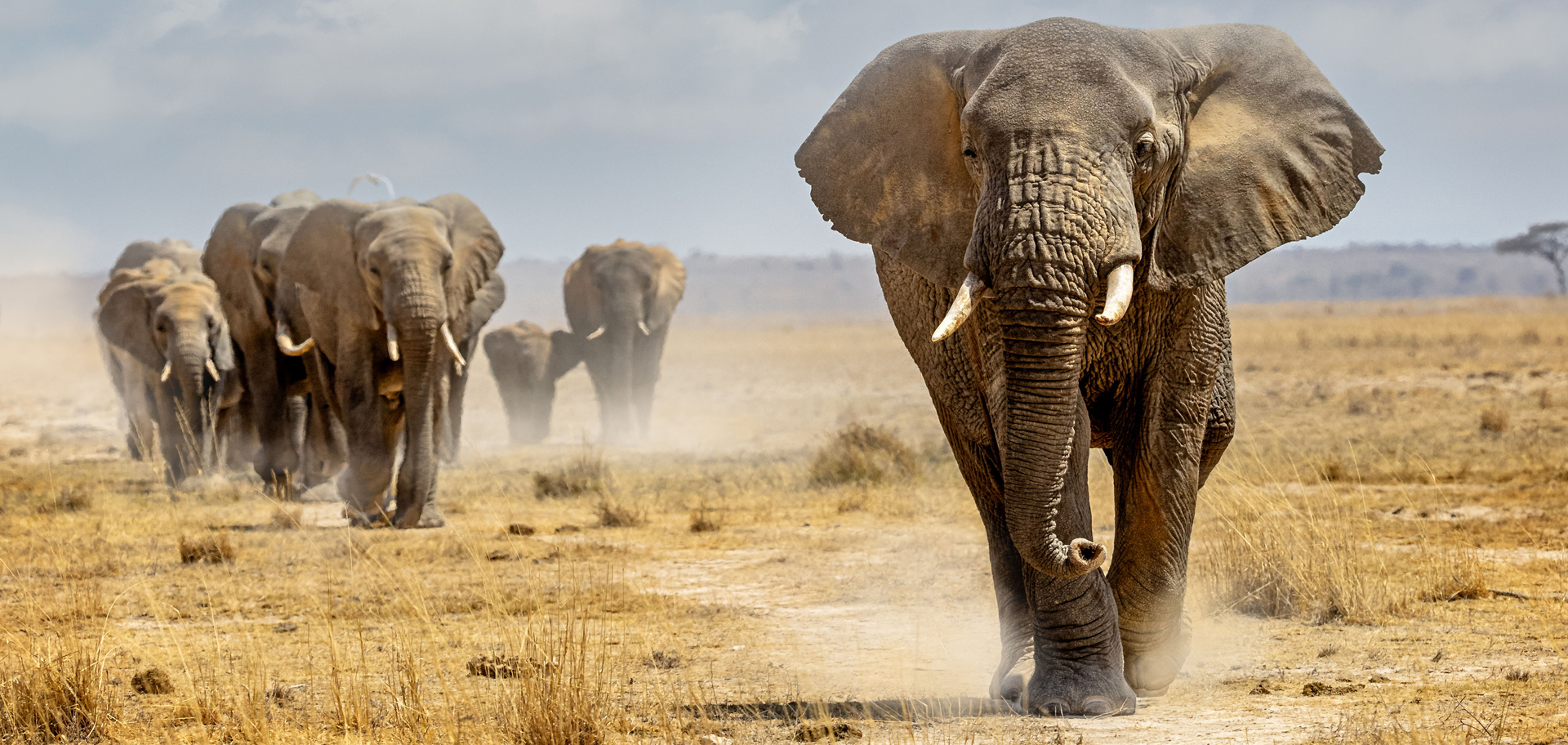
Running for a large animal, such as an elephant, is like lifting an enormous weight because their muscles are relatively weaker, and gravity demands a larger cost. As a result, as they get bigger, animals must eventually slow down.
In addition to explaining how fast animals can run, the new model may also provide critical clues for understanding differences between groups of animals. Large reptiles, such as crocodiles and lizards, are generally smaller and slower than large mammals. One possible explanation for this may be that limb muscle is a smaller percentage of reptiles’ bodies by weight, meaning that they hit the work limit at a smaller body weight, and thus must remain small to move quickly.
The model, combined with data from modern species, also predicted that land animals weighing heavier than 40 tons would be unable to move. The heaviest land mammal alive today is the African elephant at around 6.6 tons, yet some land dinosaurs, like the Patagotitan, likely weighed much more than 40 tons.
The researchers say this indicates that we should be cautious when estimating the muscular anatomy and physiology of extinct animals from data on nonextinct ones. Instead, they suggest the data indicates that extinct giants might have evolved unique muscular anatomies. The question warrants more study, such as how did massive dinosaurs manage to move?
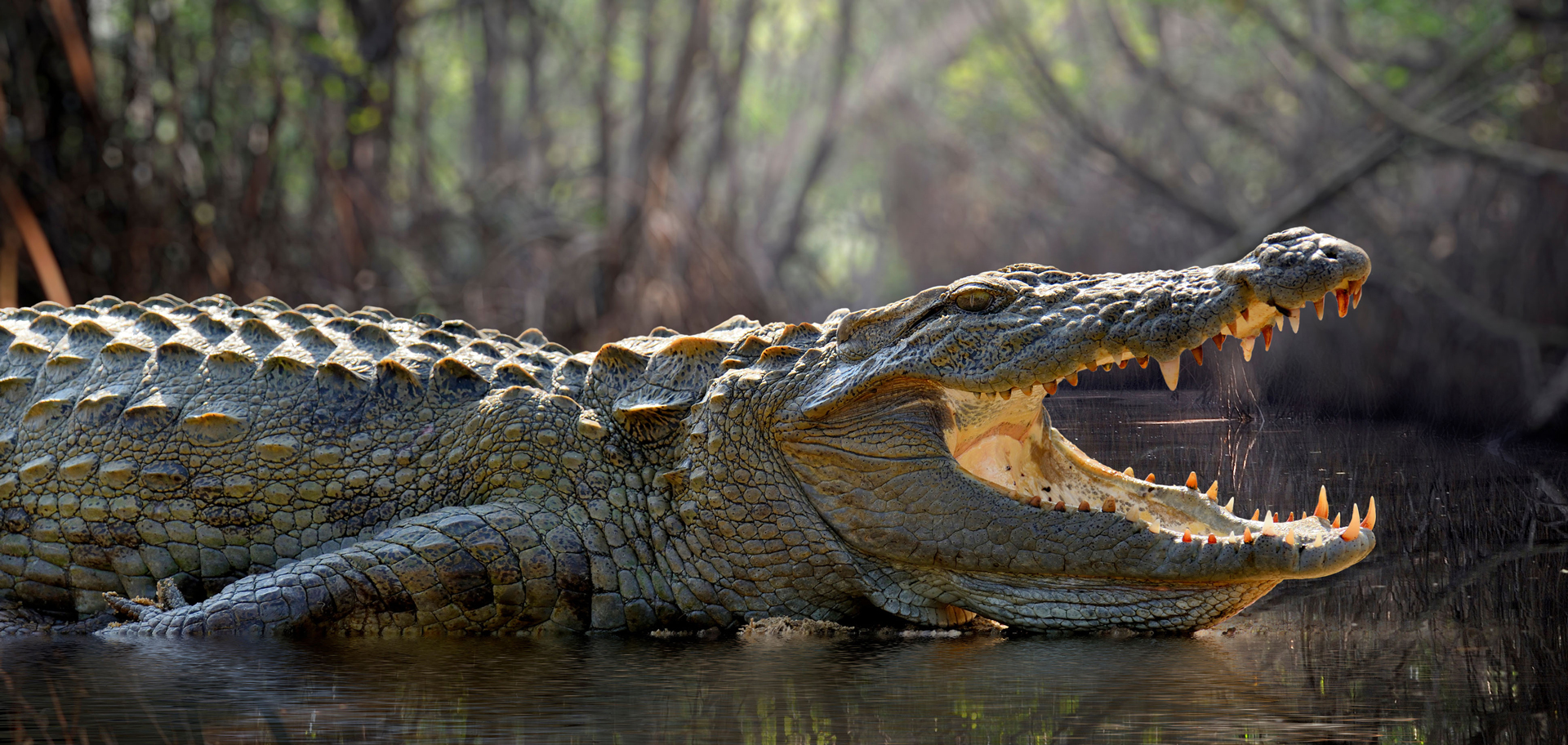
Crocodiles and other large reptiles are generally smaller and slower than large mammals. It’s theorized that limb muscle is a smaller percentage of reptiles’ bodies by weight, meaning that they hit the work limit at a smaller body weight, and thus must remain small to move quickly.
While the study looked only at land animals, the researchers will next apply their methods to animals who fly and swim. The findings could shed light on the physical principles behind how muscles evolved and could inform future designs for robots that match the athleticism of the best animal runners.
For sea turtle hatchlings, a bigger size means a faster trip to the ocean
Female sea turtles lay their eggs on a beach, cover the nest with sand and then return to the ocean, leaving them to develop and hatch on their own. From nest predators to rising temperatures, odds of survival are bleak. Even after once hatched and in the ocean, only about one in 1,000 make it to adulthood.
That’s why hatchling size matters. Larger hatchlings, which move faster, are more likely to survive because they spend less time on risky beach sands. Now, a collaborative study led by scientists from the University of Tubingen in Germany in association with Florida Atlantic University scientists delves into how fluctuating rainfall impacts the development of sea turtle hatchlings, revealing that it has a more profound effect than changes in air temperature. The research, which spans data from 37 beaches worldwide—a longitudinal study (a research design that involves repeated observations of the same variables over long periods of time) in Florida and experimental studies on Africa’s Cape Verde Islands—shows that precipitation plays a crucial role in determining hatchling body size. Rainfall cools the beach surface and enhances the moisture needed for egg development, making it a better predictor of body size than temperature.
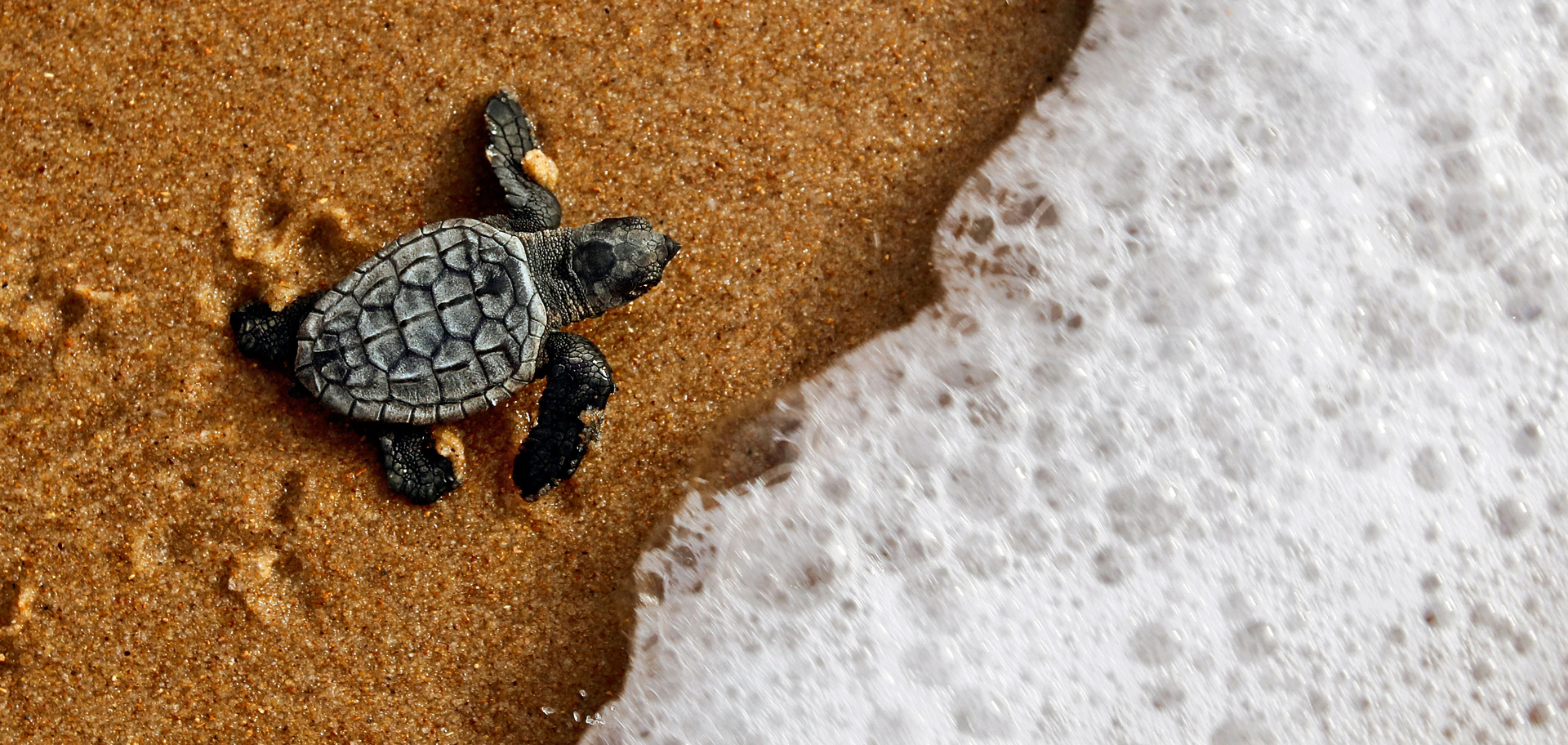
According to World Wildlife Fund, loggerheads are the most common turtle in the Mediterranean. A fundamental link in marine ecosystems, these turtles help maintain the health of coral reefs and seagrass beds. Unfortunately, climate change and tourism development threaten their nesting beaches.
But balancing moisture and temperature is vital for the health of these vulnerable sea turtles. Too much moisture can be deadly for embryos. And while cooler temperatures produce larger, heavier hatchlings with more males, warmer temperatures accelerate hatching and offer predator protection.
The study started with data from Boca Raton, Florida, that compared hatchling size (length, mass and width) with local climate factors. Next, data were collected from 19 beaches with vulnerable loggerhead turtle hatchlings and 17 beaches with green sea turtle hatchlings. A third part of the study looked at hatchlings in Cabo Verde, Africa, after a few days of rain during the dry season to see how precipitation affected their size.
In the Mediterranean, particularly on the beaches of Cyprus and Turkey, the dry season brings very little rain from the North Atlantic. For green sea turtles in these drier areas, the effects of precipitation become noticeable only after the dry season ends. In Florida, however, precipitation levels stay consistent throughout the nesting season due to local weather patterns, though droughts and heat waves typically occur in July and October.
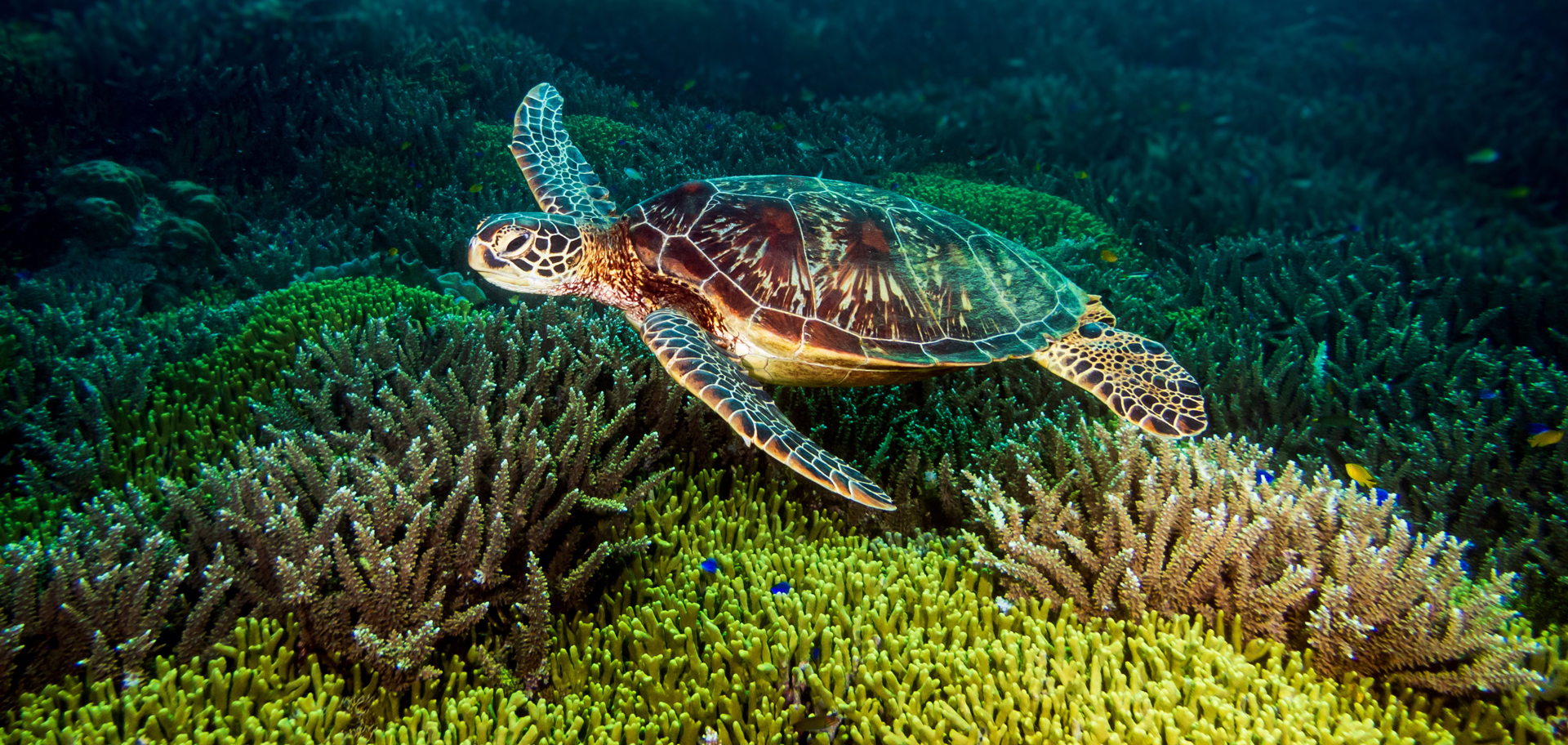
Findings from a sea turtle study highlight the need for more localized data on how regional weather influences incubation and hatchling development. This information is essential for refining conservation strategies to protect green sea turtles, such as this one, in an age of global warming.
Results of the study, published in the science journal BMC Ecology and Evolution in August 2024, reveal that the impact of rainfall varies between species. For loggerhead turtles, heavier rainfall results in hatchlings with smaller carapaces (shells) but greater weight, while green sea turtle hatchlings grow smaller carapaces without a change in body mass. For both species, though, cooler and moister conditions result in stronger hatchlings. However, rising temperatures might shorten incubation periods, and erratic rainfall can disrupt growth, potentially affecting survival.
While it’s not clear exactly how rain affects hatchling size, one idea is that rain cools the nests, which can change the temperature and impact the sex ratio of the hatchlings. This could lead to differences in hatchling size and shape, similar to how male and female hatchlings of other turtles, such as giant river turtles, have different shell shapes.
As climate change shifts rainfall patterns—making wet areas wetter and dry areas drier—the impact on sea turtle nesting sites suggests that global conservation strategies for loggerhead and green sea turtles likely need to be updated with local data. These local databases should be made more accessible and widely shared to improve our knowledge and support local conservation efforts.
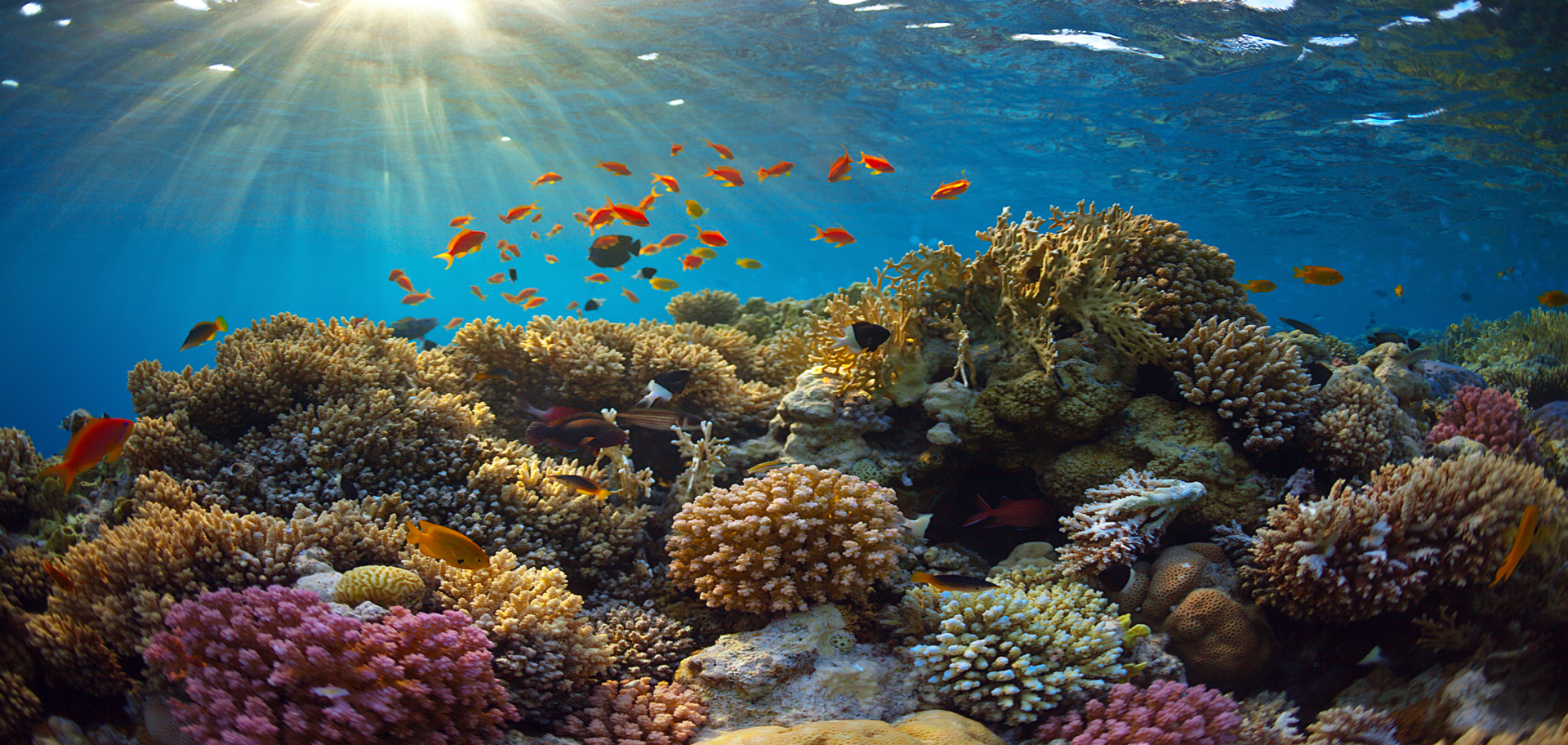
New, detailed satellite mapping is being used to inform coral reef conservation projects in Africa, Australia, Belize, Fiji, India, Indonesia, Panama, the Solomon Islands and Sri Lanka.
For coral reefs, bigger and more is better
Globally, coral reefs have declined by half since the 1950s, largely due to climate change. Current predictions for emissions of greenhouse gases place almost all reef corals on a path toward near extinction without human intervention. According to the Intergovernmental Panel on Climate Change (IPCC), human activities are estimated to have caused approximately 1.0 degrees Celsius of global warming above preindustrial levels, with a likely range of 0.8 to 1.2 degrees Celsius. Global warming is likely to reach 1.5 degrees Celsius between 2030 and 2052 if it continues to increase at the current rate. And if the world warms by 1.5 degrees Celsius relative to preindustrial times, coral reefs could decline by 70% to 90%.
But here’s some good “size” nature news: a University of Queensland-led study, the results of which were published in the science journal Cell Reports Sustainability in August 2024, shows that there is more coral reef area across the globe than previously thought, and detailed satellite mapping could help to conserve these vital ecosystems.
More than 1.5 million samples and 100 trillion pixels from the Sentinel-2 and Planet Dove CubeSat satellites were used to capture fine-scale detail on a high-resolution global map, marking the first accurate depiction of the distribution and composition of the world’s coral reefs. Scientists have now identified 134,363 square miles of shallow coral reefs, up to 65 to 98 feet deep, reversing previous estimates of shallow reefs in the world’s oceans. The researchers were also able to see what these habitats are made from: 30,888 square miles of reef have a hard bottom, where coral tends to grow, as opposed to a soft bottom, such as rubble, sand or seagrass.
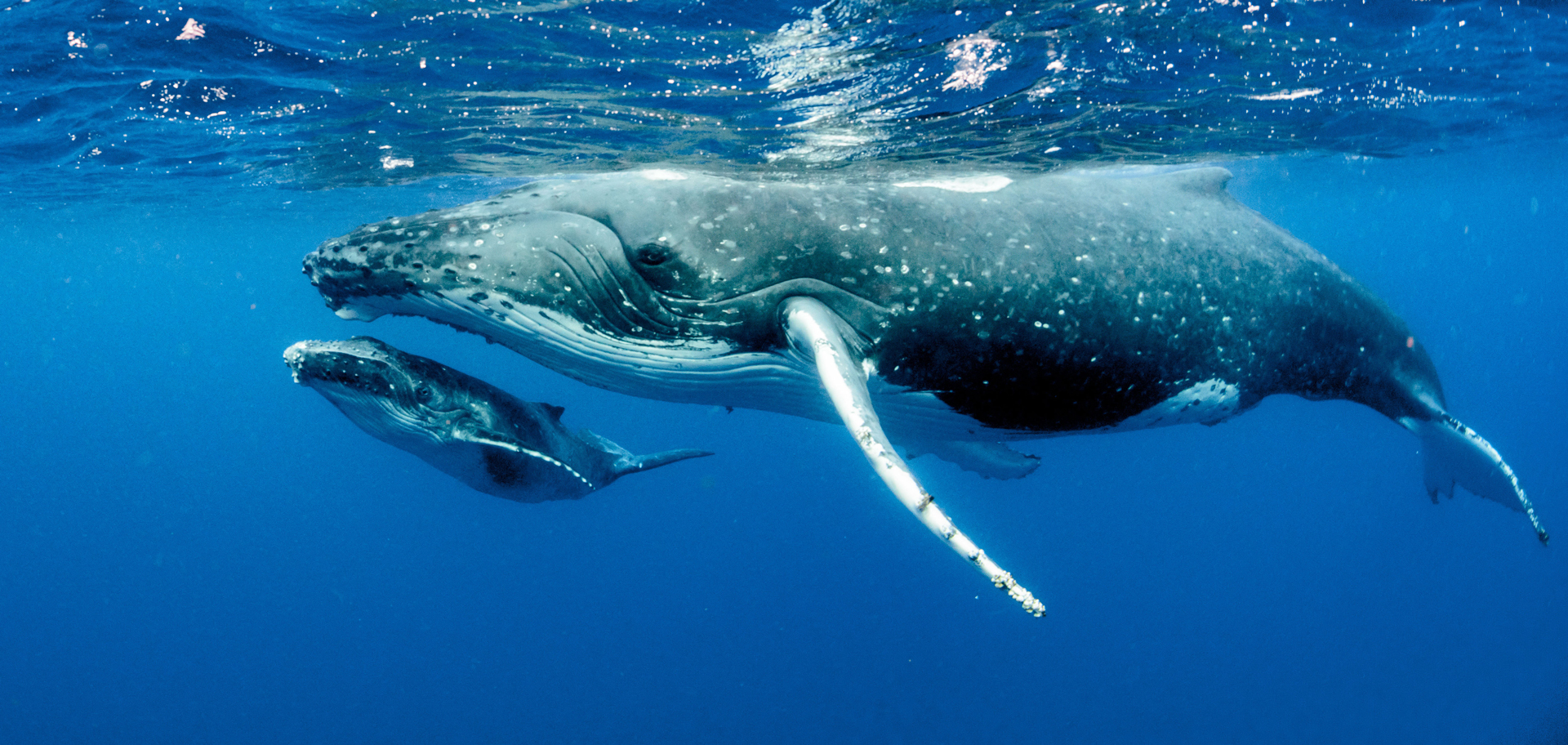
Size in nature is complex and multifaceted. Take brains, for example. Humans are a particularly extreme case; our brains are roughly seven times larger than expected for an animal of our size. Dogs are also famously large-brained and smart, as are dolphins, elephants and whales.
The reef mapping project, publicly accessible through the Allen Coral Atlas and Google Earth Engine, is a collaboration with more than 480 contributors. The details provided by these maps is already being used by conservationists, policymakers and scientists to better understand and manage reef systems and coastal and marine environments around the world.
For beings on Earth, size is complicated
Size is a complicated issue in nature; bigger isn’t always better. If you’re a mammal with a big brain and a small body, your largeness won’t much matter. In fact, if you’re a cheetah, you might be better off being medium-sized. But if you’re a sea turtle hatchling or a coral reef, being big does tip the scales in your favor.
Perhaps size in nature isn’t a “bigger is better” standard; perhaps it’s more of an “everything has got its right size” truism.
Here’s to finding your true places and natural habitats,
Candy